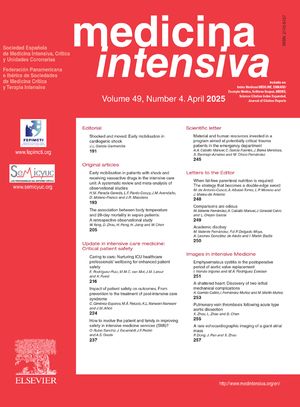
Edited by: Federico Gordo - Medicina Intensiva del Hospital Universitario del Henares (Coslada-Madrid)
Last update: February 2024
More infoThe rise of infections caused by multi-resistant gram-negative bacilli (MR-GNB), which includes carbapenems, represents one of the major current challenges worldwide. These MR-GNB include extended spectrum β-lactamase-producing Enterobacterales, derepressed AmpC-producing or carbapenemase-producing Enterobacterales as well as non-fermenting Gram-negative bacilli such as Pseudomonas aeruginosa or Acinetobacter baumannii. P. aeruginosa predominantly exhibits other resistance mechanisms different to β-lactamases such as expulsion pumps or loss of porins. A. baumannii frequently presents several of these resistance mechanisms. Mortality is high especially if empirical treatment is inadequate. In this review, treatment strategies are revised, describing the tools available to identify patients in whom empirical antibiotic treatment would be justified to cover MR-GNB, the importance of optimizing the administration of these antibiotics, as well as prevention strategies to avoid its spread from patients colonized or infected by a MR-GNB.
El aumento global de infecciones causadas por bacilos gram-negativos multi-resistentes (BGN-MR), lo cual incluye a los carbapenemes, supone uno de los grandes retos actuales en materia de sanidad. Esto incluye Enterobacterales productores de β-lactamasas de espectro extendido, productoras de AmpC desreprimida o Enterobacterales productores de carbapenemasas, así como BGN-MR no fermentadores como Pseudomonas aeruginosa o Acinetobacter baumannii. En Pseudomonas aeruginosa predominan otros mecanismos de resistencias diferentes a las β-lactamasas tales como bombas de expulsión o pérdida de porinas. A. baumannii presenta con frecuencia varios de estos mecanismos de resistencia. La mortalidad es elevada especialmente si el tratamiento empírico es inadecuado. En este capítulo se revisan las estrategias de tratamiento haciendo hincapié en las herramientas para identificar los pacientes en los que estaría justificado tratamiento antibiótico empírico para cubrir BGN-MR, la importancia de la optimización de la administración de estos antibióticos, así como las estrategias de prevención para evitar su diseminación desde pacientes colonizados o infectados por un BGN-MR.
Antibiotic resistance is one of the leading health problems we face today according to the World Health Organization (WHO). A recent report anticipates that almost 300 million people will die within the next few decades as a direct result of antimicrobial resistance. Specifically, by the year 2050, it is estimated that nearly 3 million people will die of infections caused by multi-resistant E. coli, and that infections will be the leading cause of mortality above cancer.1 In Europe, an epidemiological study conducted back in 2015 confirmed a rate of 131 infections due to multi-resistant bacteria (MRB) per 100 000 inhabitants. The mortality attributed to these infections was 6.44 patients dead per 100 000 inhabitants (attributed mortality > 33 000 people) causing the loss of 170 years of life adjusted by disability per 100 000 inhabitants.2
Although such problem affects most bacteria and other pathogens like fungi it is undoubtedly the development of resistances by gram-negative bacilli (GNB) the one that been the highest cause for alert in the scientific community. This includes extended-spectrum beta-lactamase-producing Enterobacteriaceae (ESBL), derepressed AmpC-producing Enterobacteriaceae or carbapenemase-producing Enterobacteriaceae (CPE), as well as multi-resistant non-fermenting GNB like Pseudomonas aeruginosa, and Acinetobacter baumannii.3
The overall increase of CPE is alarming and represents a growing threat for healthcare workers and the safety of the patients. Over the last few years, the epidemiological situation of CPE has become considerably worse, especially due to the fast spread of Klebsiella pneumoniae carbapenemase-producing Enterobacteriaceae (KPC), oxacillinase 48-producing Enterobacteriaceae (OXA-48) or Metalo-beta-lactamase-producing Enterobacteriaceae. Therefore, in 2015, 13 out of 38 European countries reported on the inter-region spread of CPE or an endemic situation while in 2013 only 6 out of 38 countries reported this situation.4
Also, Pseudomonas aeruginosa is still a problem, a non-fermenting GNB with high capacity to develop resistances. We should add Acinetobacter baumannii, a pathogen that basically causes nosocomial infections characterized by its great persistence and ubiquity in the environment thanks to which it can spread rapidly. Also, it has an extraordinary capacity to develop resistance to all conventional antimicrobials, and some biocides.5
Epidemiology of multi-resistance in gram-negative bacilli in the intensive care unit settingBeta-lactam resistance including carbapenems in case of Enterobacteriaceae is basically due to the expression of the enzymatic inactivation of antibiotics (beta-lactamases). However, other non-enzymatic mechanisms can be involved too (efflux pumps or porin loss). These beta-lactamases can originate from mutations in chromosomic genes, but often originate due to the horizontal transfer of mobile genetic elements through plasmids or transposons.6
However, P. aeruginosa has other predominant resistance mechanisms different than beta-lactamases like efflux pumps or loss of porins.7 A recent study conducted in Spain that analyzed 1445 clinical samples of P. aeruginosa found that only in 3.1% of the isolates 1 extended-spectrum beta-lactamase or 1 carbapenemase were found being the VIM-type the one identified most often. Nonetheless, the presence of derepressed AmpC was even more common as it was reported in 8.3% of the isolates.8 Regarding A. baumannii, it holds innate resistance mechanisms against multiple antimicrobials in its core genome. Also, the strains can easily acquire new resistance determinants through several mobile genetic elements. Almost all antimicrobial resistance mechanisms have been described including enzyme inactivation, bacterial target alteration, patency barriers for antimicrobial absorption or active efflux pumps. Regarding carbapenem-resistance mechanisms, the most common ones are the presence of carbapenemases, especially VIM-type Metalo-beta-lactamases, and D-class beta-lactamases, mainly OXA-23, and OXA-58.5,7
In any case, the rate of multi-resistant GNB (MR-GNB) is a general problem that has been gaining traction over the last few years. In a survey conducted among European intensivists back in 2017, 12.4% of the participants said that over the last 6 months they had treated, at least, 1 infection due to a resistant or almost multi-resistant bacteria to all antibiotics from the therapeutic armamentarium.9 A multicenter study conducted in 116 intensive care units (ICU) from the 5 continents analyzed the data of 1156 patients with nosocomial bacteremia (a total of 1317 pathogens were isolated) and confirmed the presence of carbapenem-resistance in 69% of Acinetobacter spp. (71.3% was categorized as extremely resistant), 38% of Klebsiella pneumoniae (71.3% was categorized as extremely resistant), 37% of Pseudomonas spp., 5.7% of Enterobacter spp., and 1% of Escherichia coli.10
In a clinical trial conducted in Spain, Italy, and Greece that randomized patients with ventilator-associated pneumonia (VAP) with a high risk of MR-GNB, 212 bacteria were isolated, mainly GNB (82%). The most common pathogens found were P. aeruginosa, A. baumannii, and K. pneumoniae. Of these, 38.1% were carbapenem-resistant while 12.4% were colistin-resistant.11 Out of the 65 isolates in respiratory sample of A. baumannii of this clinical trial all but 2 (97%) were carbapenem-resistant and always associated with 1 acquired carbapenemase, the OXA-23 mainly (80%). We should mention that the rate of colistin-resistance in these A. baumannii was 47.7%.12
Prognosis of infections due to multi-resistant gram-negative bacilliSeveral authors have demonstrated that these infections have high morbidity and mortality rates especially when they affect critically ill patients. A study conducted on the prevalence and prognosis of infections at the ICU setting (62% due to MR-GNB) confirmed that the mortality rate of infected patients more than doubled that of uninfected patients (25% vs 11%). In this study, after adjusting for several confounding variables, the infections due to beta-lactamase-resistant Klebsiella spp. (including carbapenems), and carbapenem-resistant A. baumannii were identified as independent factors of in-hospital mortality.13
The mortality rate of bacteremia due to carbapenem-resistant GNB in a cohort of critically ill patients doubled that of the control group that was bacteremia-free (49.3% vs 25.6%) being an independent risk factor for mortality after adjusting for several confounding variables. Also, it is associated with an extended length of stay (median of 30 days vs 12 days) with the corresponding associated higher hospital costs.14 Also we should mention that the mortality rate due to CPE-induced bacteremia is, after adjusting for the possible confounding variables, 4 times higher compared to the mortality rate associated with episodes of CPE-induced bacteremia, but with other resistance mechanisms since they did not produce carbapenemase.15
A multicenter trial conducted in 137 Italian ICUs compared the prognostic impact of infections due to multisensitive K. pneumoniae, ESBL-producing K pneumoniae, and carbapenem-resistant K. pneumoniae (the main infection isite was pulmonary followed by intra-abdominal). Infections due to carbapenem-resistant K. pneumoniae had a higher mortality rate of 20% compared to the predictive mortality rate, which was especially evident in patients with high risk of mortality (predicted risk > 32%) which was not reported in the case of carbapenem-sensitive isolates, but still ESBL-producing.16
Risk factorsNew epidemiological studies have demonstrated that there is a direct association between the use of antibiotics and the development of resistance to these antibiotics by GNB. This has been demonstrated in the case of CPE,17,18Pseudomonas,19–21 and Acinetobacter spp.19,22–24 It is well known that for every additional day of exposure to antipseudomonal beta-lactam antibiotics, the risk of appearance of a new resistance increases by 2% for meropenem and up to 8% for cefepime or piperacillin/tazobactam.25 However, the mechanisms to develop resistance by GNB are complex, and this correlation between the use of certain antibiotics, and the appearance of resistance does not always occur.26
On the other hand, the profile of patients who develop infection due to MR-GNB includes vulnerable patients with extended length of stay, and previous use of broad-spectrum antibiotic therapy. The most commonly risk factors are shown on Table 1.
Most commonly identified risk factors for infections due to multi-resistant gram-negative bacilli.
- Old age. |
- Previous colonization (especially by A. baumannii, and ESBL-producing Enterobacteriaceae and carbapenemases) |
- Institutionalized patient |
- Underlying severity |
- Immunosuppression |
- Emergency admission |
- Admission due to respiratory failure |
- Previous antibiotic therapy (previous use of carbapenems for carbapenem-resistant GNB) |
- Multiple invasive procedures |
- Long ICU stays |
ESBL, extended-spectrum beta-lactamases; GNB, gram-negative bacilli; ICU, intensive care unit.
The 2 key aspects in the success of antibiotic therapy regarding severe infections are early and proper treatment, and the proper dosing to achieve therapeutic levels especially at the infection site.
In a multicenter study that analyzed 1156 episodes of hospital-acquired bacteremias, the rate of MRB and extremely resistant bacteria was 48% and 21%, respectively. In the multivariant model, isolating the MRB and the uncontrolled focus were independent predictors of the 28-day mortality rate while early treatment was identified as a 347protector of mortality.10 The time elapsed until the proper antibiotic therapy was used was an independent risk factor for mortality in patients with bacteremia due to KPC-producing K. pneumoniae. The median time elapsed from the beginning of the proper treatment until blood culture drawn was 8.5 h in patients who survived, and 48 h in those who died within the next 30 days.27
Identification of patients eligible for evidence-based antibiotic therapy to cover multi-resistant gram-negative bacilliRegarding the selection of the appropriate antibiotics that should be used in evidence-based treatment, the difficulty here is identifying those patients who may suffer an infection due to MR-GNB and use active antibiotics against such pathogen. Therefore, the clinician has 3 possible tools at his disposal: knowledge on whether the patient is colonized by some of these MR-GNB, the microbiological techniques of rapid diagnosis, and artificial intelligence-based algorithms.
The association between the colonization of the digestive tract by certain types of MR-GNB and further development of invasive infections due to such pathogen, especially pneumonias or bacteremias is well established. This has been confirmed for A. baumannii,28–31 and ESBL-producing Enterobacteriaceae,32–38 AmpC-producing Enterobacteriaceae,39,40 and several types of carbapenemase-producing Enterobacteriaceae.41–44 The capacity to produce disseminated infections seems higher in cases of colonization by KPC-producing strains compared to cases of colonization by Metalo-beta-lactamase or other beta-lactamase-producing Enterobacteriaceae.44,45
Table 2 summarizes the main findings of studies that analyzed the predictive value of colonization by ESBL-producing Enterobacteriaceae to identify the pathogen causing the VAP. The positive predictive value (PPV) is always lo and, in any case, < 50% while the negative predictive value (NPV) is very high in all studies (> 90%). It has not been defined whether these patients colonized by MR-GNB should receive evidence-based antibiotic therapy to cover such GNB in case of developing the infection. This can lead to overusing a certain group of antibiotics, which has been confirmed in the case of patients colonized by ESBL-producing Enterobacteriaceae who develop VAP, which led to overusing carbapenems in evidence-based treatment despite the low rate of VAP confirmed by these pathogens.46 No studies have been published to this date on the increased use of active antibiotics against CPE, although this is what will probably happen if the evidence-based use of these antibiotics becomes popular in patients colonized by such pathogens who end up developing an infection. In any case, the guidelines published by the British Society for Antimicrobial Chemotherapy on the management of infections due to MR-GNB considers that only patients with risk factors and colonized with MR-GNB who develop an infection should receive active antibiotics to fight against such pathogens.47
Studies that assessed the predictive value of colonization by ESNL-producing Enterobacteriaceae for the identification of the pathogen causing VAP.
Author (ref)/design | Pathogen analyzed/frequency | No. of patients included/No. of VAPs/ No. of VAPs due to GNB analyzed | PPV/NPV | Comments |
---|---|---|---|---|
Bruyère et al.32/retrospective | ESBL-producing Enterobacteriaceae/At admission and on a weekly basis | 3439/587/20 | 41.5%/ 99.4% | |
Razazi et al.34/prospective | ESBL-producing Enterobacteriaceae/At admission and on a weekly basis | 6303/843/157 | Unreported | Colonization with E. cloacae or K. pneumoniae was a risk factor for VAP compared to E. coli or E. aerogenes |
Houard et al.35/retrospective | ESBL-producing Enterobacteriaceae/At admission and on a weekly basis | 1570/469/43 | 43.6%/97.3% | |
Massart et al.38/retrospective | ESBL-producing Enterobacteriaceae/At admission and on a weekly basis | 3250/205/15 (8 VAP, and 7 PB) | 31.6%/95.2% | Combined analysis of VAP and PB |
Manquat et al.40/retrospective | ESBL-producing Enterobacteriaceae and AMPC-H-producing Enterobacteriaceae/At admission and on a weekly basis | 3228/468/39 ESBL, and 36 AMPC-H | 14.8%/98.8% for E-ESBL/20.3% and 98% for AMPC-H | The method used to isolate the rectal sample is specific for E-ESBL, not for AMPC-H, which may have underestimated the actual number |
AMPC-H, AmpC hyperproducer; ESBL, extended-spectrum beta-lactamases; GNB, gram-negative bacilli; NPV, negative predictive value; PB, primary bacteremia; PPV, positive predictive value; VAP, ventilator-associated pneumonia.
Other tools available to help in the selection of the initial treatment are rapid microbiology detection tests. Traditional microbiological tests take, at least, 24 h to 48 h to identify microorganisms, and 48 h to 72 h to determine the antibiogram. To solve this problem, different rapid diagnostic techniques (from 1.5 h to 6 h) have been developed based on the amplification of nucleic acids that allow rapid identifications of microorganisms, and that can also detect several resistance genes (eg, those coding ESBL or carbapenemase). However, if the mechanism of resistance to beta-lactam antibiotics—including carbapenems—is different from an inactivating enzyme (overexpression of efflux pumps, loss or mutation of porins…) they cannot be detected through theses molecular systems. Also, these tests are expensive (> euro100 per test) and should be performed together with conventional cultures that, to this date, they still can’t replace.3
Finally, artificial intelligence-based algorithms can be a useful tool to identify patients who may have an infection due to a multi-resistant bateria.48 Therefore, a recent study develops an algorithm that, with a very modest PPV (30%), but with a very high NPV (99%) can identify patients with infection due to carbapenem-resistant GNB.49
Optimization of antibiotic therapy to improve prognosisStandard dose regimens are often unsuitable for critically ill patients. There are 2 main differences in the pharmakokinetic/pharmacodynamic (PK/PD) parameters between critically ill patients and the rest of the patients: the increased volume of distribution and the increased clearance of antibiotics excreted through the kidneys in hyperfiltrating or patients with renal hyperclearance. These variables—together with the minimum inhibitory concentration of pathogens involved and the precocity of treatment—are key to achieve clinical healing (Fig. 1). A study conducted among 285 critically ill patients (1660 determinations of creatinine clearance) revealed that 65% of the patients increased their renal function—defined by creatinine clearance levels of 130 mL/min/1.73 m2—within the first week after hospitalization. This finding is particularly worrying because most antimicrobial regimens in the ICU setting are based on beta-lactam antibiotics that clear out predominantly through the kidney, which makes that most patients with renal hyperclearance cannot achieve therapeutic concentrations to treat GNB-induced infections with the dosage recommended on the drug label.50 This also affects new beta-lactam antibiotics recently introduced in the therapeutic armamentarium that are active against MR-GNB like ceftolozane-tazobactam51 or cefiderocol.52
We should mention that in a recent study of 577 adult patients with infections due to KPC-producing K. pneumoniae—391 of them with bacteremia—treated with ceftazidime-avibactam the antibiotic dosage was adjusted based on the renal function, and it turned out to be an independent risk factor for mortality after 30 days while its use in extended perfusion was identified as a protective factor.53
Role of antibiotic optimization programsAntibiotic optimization programs (AOP) were created to improve the use of antimicrobial agents, increase their efficacy, and reduce adverse events, infections due to Clostridium difficile, and the appearance of antimicrobial resistance. Also, as secondary endpoints, to reduce healthcare costs and avoid unnecessary treatments and complications.
AOP have proven to be an efficient strategy to reduce the rates of MRB in all healthcare settings: at community, hospital, and specifically ICU level. A meta-analysis that included 24 studies (only 3 were randomized clinical trials) demonstrated a lower use of antimicrobials with the corresponding lower cost and shorter use time. Also, procedures that went on for over 6 months were associated with lower rates of antimicrobial resistance.54
In Spain, 2 studies published reported on the impact of one multidisciplinary AOP implemented in the ICU setting and led by intensivists. The first one proved that this program achieved a significant reduction in the use of antimicrobials at the ICU setting without affecting mortality, although it did not reduce the isolation of multi-resistant bacteria.55 The other study that compared 2 different time periods (2007 to 2010 vs 2011 to 2015) proved that a significant reduction in the number of patients who received one or more antibiotics was achieved (assessed by dose defined on a daily basis and on the course of treatment), and savings were close to euro1 000 000 since the implementation of the AOP. We should mention that the overall mortality rate was not affected. Also, the rate of infections due to multi-resistant bacteria dropped significantly from affecting 15.8% to 32,4% of the patients in 2007 and 2008 down to 2.8% and 3.3% of the patients in 2014 and 2015.56
The implementation of an AOP in 2 Dutch ICUs also confirmed that a significant reduction in the use of antibiotics was achieved accompanied by a lower rate of catheter-induced bacteremia due to MR-GNB.57 An intervention study designed to reduce the duration of antibiotic therapy at the ICU setting proved that 10 months after implementing the AOP, a statistically significant reduction in the number of infections due to imipenem-resistant A. baumannii and K. pneumoniae was achieved.58 Finally, we should mention that a program to review antibiotics that have the greatest ecological impacts (quinolones, carbapenems, and cephalosporines) plus feedback from the prescribers translated into a lower rate of resistances in P. aeruginosa, and in the rate of AmpC-hyperproducing Enterobacteriaceae.59 We should mention that all these studies confirm that mortality does not change after the implementation of these AOP, which are associated with a lower use of antibiotics and, in several studies, with lower rates of resistances, especially in GNB.
One of the strategies anticipated in these AOP is therapeutic de-escalation. Such strategy consists of early changes to evidence-based wide-spectrum antimicrobial therapy to prevent the appearance of antimicrobial resistance by reducing the overall exposure to wide-spectrum antibiotics. Therapeutic de-escalation has been proven a safe practice that is performed when downgrading combined therapy to monotherapy or reducing the antimicrobial spectrum when only one wide-spectrum antimicrobial agent was used based on the evidence available.60,61 Early withdrawal of evidence-based therapy when diagnosis is achieved that the clinical signs that triggered the use of antibiotics were not infection-like is also a good clinical practice that should always been performed, although conceptually speaking it is not considered therapeutic de-escalation.62 Also, by reducing the number of antibiotics administered, de-escalation is also associated with less side effects, and eventually lower costs.
Many clinicians are still reluctant to reduce the spectrum of evidence-based broad-spectrum antibiotic therapy even when the patient’s progression is favorable. To this date, all studies agree that therapeutic de-escalation is safe. Despite this data, the rate of antibiotic de-escalation is still very low. In an observational, multinational study recently published conducted between October 2016 and 2018—the DIANA trial—the rate of evidence-based antibiotic therapy de-escalation was only 16%.63 In this study, the appearance of multi-resistant pathogens reported between days 2 and 28 of follow-up was lower compared to the group where therapeutic de-escalation was implemented in patients in whom evidence-based therapy was kept with a strong statistically significant tendency (7.5% vs 11.9%; P = .052).
On the other hand, it has been demonstrated that a restrictive strategy consisting of the use of antibiotics only after microbiological identification (except for cases of septic shock, acute respiratory failure or meningitis) plus an intervention aimed at shortening the course of antibiotic therapy reduced the rate of ICU-acquired ESBL-producing Enterobacteriaceae significantly, as well as the rate of infections due to these pathogens.64 We should mention that the unadjusted crude mortality rate at the ICU setting was significantly lower with the restrictive strategy compared to that from the group on conventional treatment (22.5% vs 28.6%; P < .01).
PreventionPrevention and control measures are effective to reduce the transmission of MR-GNB and are based on putting barriers to the different ways of transmission by using infection control measures.65 These measures should not only be applied to patients with confirmed infections, but also—and with the same intensity—to patients colonized by MR-GNB. Therefore, the identification—at ICU admission and during the ICU stay—of patients colonized by MR-GNB through active detection strategies is essential to reduce the spread of transmission as recommended by the SEMICYUC Zero Resistance project.66
Physical contact through the hands of people (whether healthcare workers of the family members) or else through medical equipment (phonendoscope, ultrasound machine…) or assistance gear (cranes, harnesses, …) are the main ways of transmission of MR-GNB. These isolation measures include the systematic use of standard precautions (hand hygiene, gloves, scrubs, eye protection), as well as precautions based on the mechanism of transmission (contact, droplets, airborne).
In-hospital water and the different medical equipment available that use water can be a reservoir of pathogens and are associated with healthcare. Several species of clinically significant MR-GNB including Enterobacteriaceae, A. baumannii and P. aeruginosa, are well adapted to colonize the biofilm of water conduction systems, and their presence in such systems has been associated with sporadic infections and outbreaks in critically ill patients.67–70 The strategies aimed at eliminating these reservoirs or using water for the patients’ hygiene have proven effective to eliminate the outbreaks.71
When there is an outbreak of MR-GNB a thorough analysis should be performed to establish the sources, reservoirs, and mechanisms of transmission, as well as to determine what the most effective control measures are when an outbreak occurs. Contention team should define what new biological and environmental samples should be taken for their research. Molecular typing techniques should always be used to determine whether there is an actual genetic correlation between two and more isolates of the same species.72 The results of the analysis of a nosocomial outbreak are shown on Table 3.
Study endpoints of a nosocomial outbreak due to MR-GNB
- Know the extent of the outbreak: number of patients infected, units involved, patient’s disease progression |
- Determine the source of the origin |
- Identify the ways of transmission and contagion |
- Identify the presence of beta-lactam inactivating enzymes |
- Cut out the spread of the infection |
- Avoids similar situations in the future |
MR-GNB, multi-resistant gram-negative bacilli.
A recent survey conducted among European health workers directly involved in infection management identified 3 critical areas to achieve implementation and compliance: 1) the amount of personnel dedicated to infection control, 2) the availability of educational programs y, and 3) the amount of clinical personnel available.73
Finally, we should mention that to fight the problem of multi-resistance in GNB action in different settings is required this being more effective that acting on one of the factors involved alone. Therefore, a combined strategy that combines standard precautions (hand hygiene, physical contact avoidance), existence of an antibiotic optimization program, environmental cleaning (all surfaces and medical equipment should be disinfected), and control of the different foci involved (bath or daily cleaning with chlorhexidine) has proven effective to reduce the rate of MR-GNB substantially.74 However, we should mention that by itself a daily program of disinfection with chlorhexidine at 2% failed to reduce the rate of infections due to MR-GNB in adult critically ill patients.75
ConclusionsThe increased rate of infections due to MR-GNB at all levels of the healthcare system, but especially the ICU setting, plus its high associated morbidity and mortality rates requires fast and coordinated action from all public agencies, the pharmaceutical industry, and the healthcare workers to prevent infections caused by these multi-resistant pathogens. Also, it requires that the diagnosis of these infections should be faster, and new active molecules that should prove their efficacy in well-designed clinical trials should be developed as well. Similarly, old, and new antibiotics alike should be optimized to increase their efficacy and reduce the rate of adverse events. All of it should be complemented with the training of intensivists who should be familiar with the management of these infections of great complexity since they affect critically ill patients. Also, they should collaborate and lead studies to solve the clinical questions associated with the management of these infections that can vary depending on the patient’s clinical situation, site of infection, target pathogen or resistance mechanisms involved.
FundingNone whatsoever.
Conflicts of interestJosé Garnacho-Montero participated in training activities sponsored by MSD, Shionogi, and Pfizer. Rosario Amaya-Villar participated in training activities sponsored by MSD, and Pfizer.
Please cite this article as: Garnacho-Montero J, Amaya-Villar R. El problema de la multi-resistencia en bacilos gram-negativos en las unidades de cuidados intensivos: estrategias de tratamiento y prevención. Med Intensiva. 2022;46:326–335.